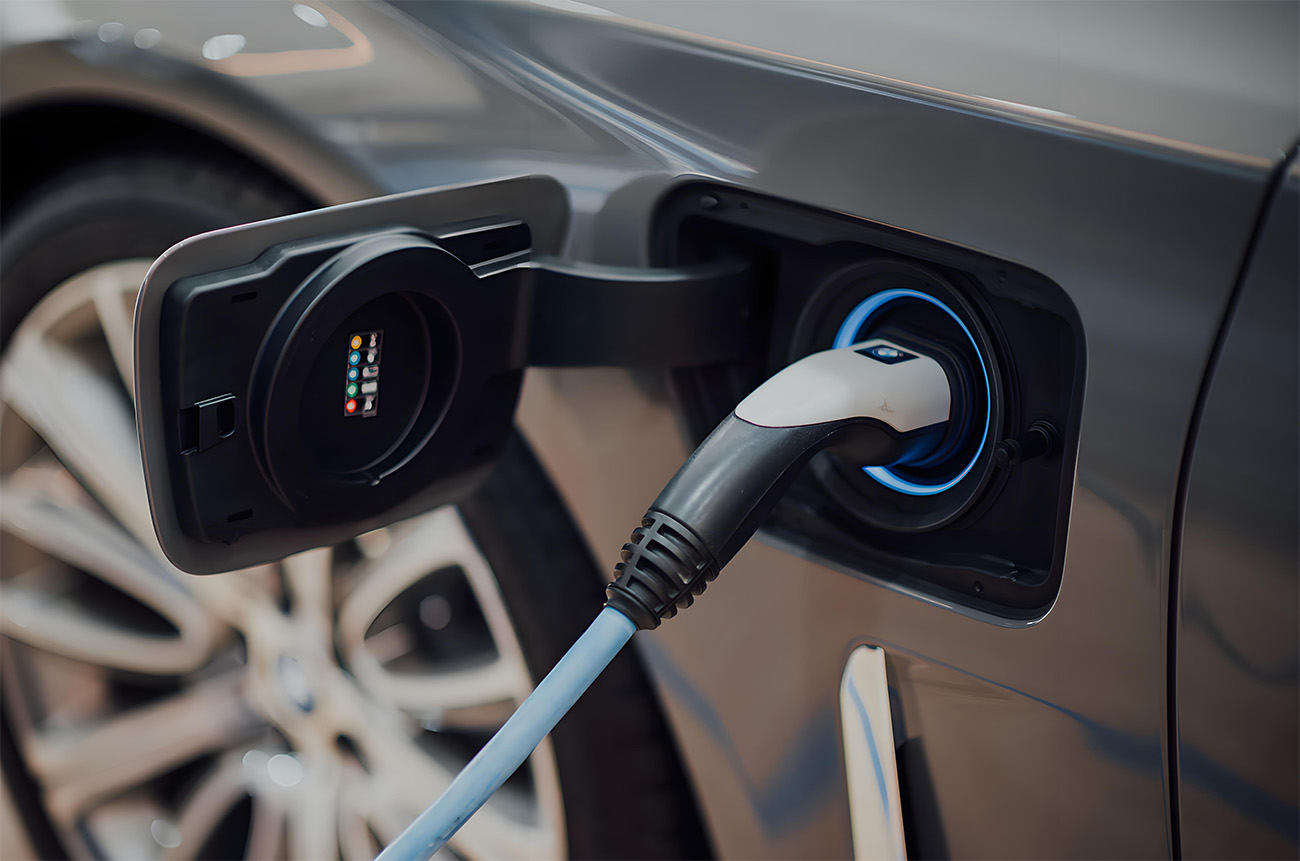
With the tightening of global CO2 emission standards, the demand for charging surpasses the supply capacity of DC fast charging stations (Level 3), leading to the emergence of Onboard chargers.
Onboard chargers (OBCs) address a critical issue in electric vehicles (EVs). They convert AC power from the grid into DC power suitable for battery charging, enabling
EV charging. With the increasing diversity of EV designs, architectures, and sizes being introduced annually, the implementation of OBCs has become increasingly complex.
Furthermore, as the industry shifts toward higher-voltage batteries for faster charging, bidirectional charging is becoming more prevalent. System designers face critical decisions regarding OBC topology and material selection.
The AC power from the grid passes through an electromagnetic interference (EMI) filter to eliminate external "noise" and prevent the OBC from feeding noise back into the grid. Then, the power enters the first of two main stages of the OBC, the Power Factor Correction (PFC) stage. The PFC stage converts AC power to DC power while significantly reducing phase distortion in the input voltage and current waveforms. This step achieves a power factor greater than 0.9 to minimize reactive power injected into the grid. Next, the current enters an isolated DC-DC converter that matches the output voltage and current to the battery's state of charge, ensuring current isolation between input and output.
Introduction to Silicon Carbide (SiC) and IGBT
SiC
Silicon carbide (SiC) is an inorganic compound with the chemical formula SiC. It is produced by high-temperature smelting in resistance furnaces using raw materials such as quartz sand, petroleum coke (or coal coke), and wood chips (salt is added in the production of green SiC). SiC is a semiconductor that occurs naturally as the extremely rare mineral moissanite. Since 1893, it has been mass-produced as powders and crystals for applications like abrasives. Among advanced non-oxide refractory materials such as C, N, and B, SiC is the most widely used and cost-effective, often called carborundum or refractory sand.
In industrial production in China, SiC is divided into black and green SiC, both hexagonal crystals.
IGBT
The Insulated Gate Bipolar Transistor (IGBT) combines the advantages of power transistors (Giant Transistor—GTR) and power MOSFETs. It is widely used and exhibits excellent characteristics. IGBT is a three-terminal device with a gate, collector, and emitter. As a MOS-based bipolar device, IGBT merges the high-speed characteristics of MOSFETs with the low resistance of bipolar devices.
IGBTs are commonly used in applications requiring voltages above 600V, currents above 10A, and frequencies above 1kHz. They are widely used in industrial motors, small-capacity civil motors, converters (inverters), camera flash controllers, induction heating devices, and rice cookers. Depending on the packaging, IGBTs are divided into two main types:
Encapsulated single-package three-terminal types (e.g., TO-3P to small surface-mounted versions).
Module types that pair IGBTs with FWDs (Freewheel Diodes) in groups of two or six for industrial applications.
Evolution of IGBT
IGBT is the natural evolution of vertical power MOSFETs for high current, high voltage, and fast terminal devices. Although newer power MOSFET generations have significantly improved RDS(on) characteristics, power conduction losses are still higher than those of IGBT at high levels.
Structural Comparison
SiC Material Characteristics:
High Breakdown Electric Field Strength: SiC’s breakdown field strength is 10 times that of silicon (Si), enabling operation at higher voltages without breakdown.
Wide Bandgap: SiC’s bandgap is three times that of Si, ensuring stability in high-temperature environments.
High-Temperature Stability: SiC devices can operate at higher temperatures than Si devices, reducing cooling requirements and system costs.
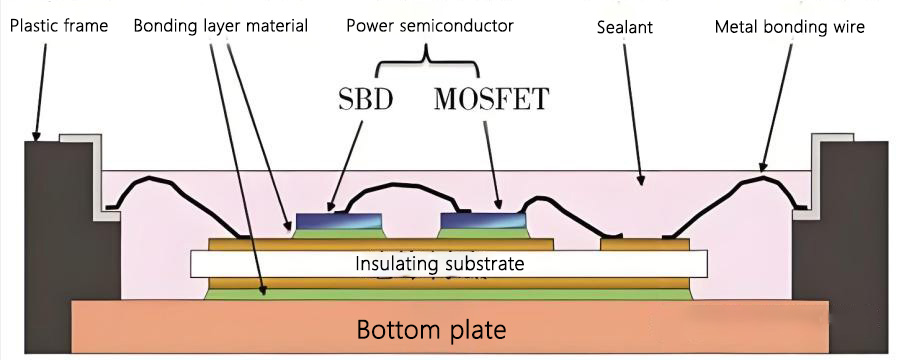
IGBT Structural Characteristics:
Composite Structure: Combines the characteristics of MOSFETs and bipolar transistors.
High Voltage Endurance: Achieves low conduction resistance under high voltage conditions via conductivity modulation.
Voltage Control: Operates via voltage-controlled switching like MOSFETs, featuring high input impedance.
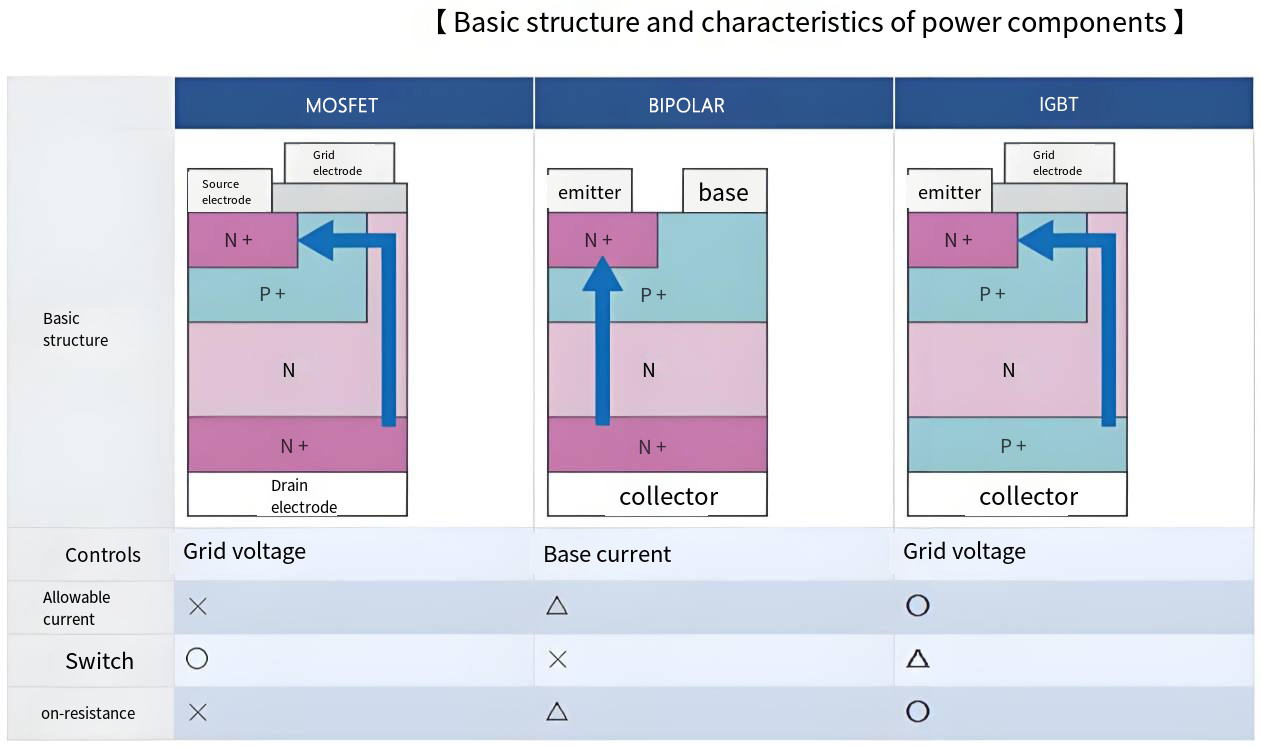
Performance Comparison
Switching Speed and Efficiency
SiC outperforms IGBT in high-speed switching, achieving switching frequencies of several hundred kHz. This makes SiC ideal for high-frequency applications like OBCs, reducing EMI and transformer size/weight. Conversely, IGBT has slower switching speeds, leading to higher switching losses and efficiency drops in high-frequency applications.
Energy Loss
SiC has lower conduction resistance and switching losses, reducing overall energy consumption. This is especially advantageous for prolonged charging or high-power scenarios, significantly improving charger efficiency while lowering thermal management requirements.
Thermal Management
SiC’s higher thermal conductivity and temperature tolerance allow operation under extreme conditions, potentially eliminating some cooling components and increasing power density. In contrast, IGBT requires more complex heat dissipation designs, increasing system size and weight.
Application Scenarios
SiC MOSFET
SiC MOSFETs are ideal for high-power, high-frequency applications, offering superior performance for 800V EV systems. They are well-suited for luxury or performance EVs requiring efficient and compact OBC designs.
IGBT
Despite higher losses at 11kW and 22kW levels, IGBTs are suitable for cost-sensitive mid-range EVs and low-frequency applications prioritizing affordability over efficiency.
Si SJ MOSFET
These are suitable for power levels below 7.2kW, and Vienna configurations can improve performance at 11kW and 22kW levels.
Summary
Selecting the right materials and topologies for OBCs is critical to optimizing charging performance and efficiency. SiC MOSFETs excel in high-efficiency, high-voltage applications, while IGBT offers cost-effective solutions for lower-voltage systems. By understanding the advantages and use cases of various components, designers can make informed decisions to enhance the overall performance of EV charging solutions.
From SiC MOSFETs to circuit protection,
Evlithiumcharger on-board charging solution contains all the components needed for a reliable, robust on-board charger design.